Crinoidea
Sea lilies and feather stars
William I. Ausich and Charles G. Messing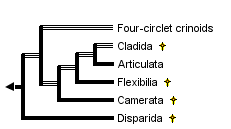


This tree diagram shows the relationships between several groups of organisms.
The root of the current tree connects the organisms featured in this tree to their containing group and the rest of the Tree of Life. The basal branching point in the tree represents the ancestor of the other groups in the tree. This ancestor diversified over time into several descendent subgroups, which are represented as internal nodes and terminal taxa to the right.
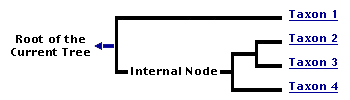
You can click on the root to travel down the Tree of Life all the way to the root of all Life, and you can click on the names of descendent subgroups to travel up the Tree of Life all the way to individual species.
For more information on ToL tree formatting, please see Interpreting the Tree or Classification. To learn more about phylogenetic trees, please visit our Phylogenetic Biology pages.
close boxIntroduction
Although crinoids are the least understood of living echinoderms, their skeletal remains are among the most abundant and important of fossils. They appeared during the Lower Ordovician and underwent several major radiations during the Paleozoic Era. Crinoids were major carbonate producing organisms during the Paleozoic and Mesozoic. In fact, in many Paleozoic and Mesozoic settings entire carbonate shelves were composed predominantly of crinoidal remains (Ausich 1997). In such examples, the enormous volume of skeletal material controlled the sedimentary environment. Short stratigraphic ranges of many species make them important as at least local biostratigraphic index taxa. The broad morphological diversity of crinoids includes forms characteristic of specific habitats and oceanographic conditions. More than 5000 fossil species have been described.
A persistent, traditional view treats living crinoids as chiefly deep-sea organisms, relicts of their opulent Paleozoic past, holding off final extinction in remote abyssal habitats. This view is generally applied to stalked crinoids, or sea lilies, as typical of the entire group, because they most closely resemble their fossil forebears. It is true that the approximately 80 extant species of stalked crinoids are chiefly restricted to depths greater than 200 m (the shallowest occurs in 100 m). However, 85% of extant crinoids (approximately 540 named species) are unstalked feather stars, or comatulids, the products of a continuing post-Paleozoic radiation (Meyer & Macurda 1977). Comatulids are a monophyletic clade classified within the subclass Articulata (Simms 1988). About 65% of living comatulids occur at shelf depths (<200 m). In the tropical Indo-West Pacific, the richest region, single reefs may support as many as 50 species, almost as many as recorded for any individual fossil assemblage. Here, abundance and diversity reach 115 specimens and 12 species per m2, respectively (Messing 1994). Although far fewer comatulid species exist in cold waters, local abundance may be much greater.
All crinoids are passive suspension feeders. They produce no feeding/respiratory current but, rather, rely on extrinsic, ambient water movement. In extant crinoids, the food-gathering apparatus functions as follows: each featherlike arm that radiates from the central body bears an open ambulacral groove bordered by triads of fingerlike podia, or tube feet, which are terminal extensions of the water vascular system (see figure below). The longest tube foot in each triad, 0.43-0.85 mm in length, is held out at a right angle and flicks passing food particles into the groove. After a food particle is captured by a crinoid, the shortest tube foot wraps it in mucous secretions; ciliary tracts on the groove floor then transport it toward the mouth. In living crinoids, food particle size ranges from about 50 to 400 µm. Diets include a variety of protists (e.g., diatoms and other unicellular algae, foraminiferans, actinopods), invertebrate larvae, small crustaceans, and detrital particles.

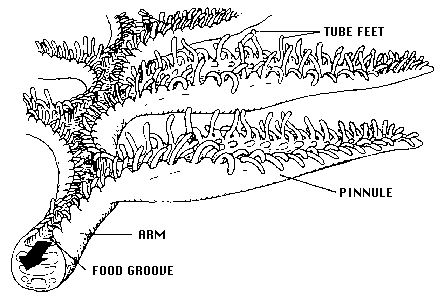
Arm and ambulacrum morphology of a living crinoid (from Messing, 1987, copyright © 1987 Charles Messing)
Characteristics
Crinoids are pentamerous, stalked echinoderms with a cuplike body bearing five usually branched and commonly featherlike arms (see figure below). Most of a crinoid's body consists of an endoskeleton composed of numerous calcareous pieces, called plates or ossicles. The visceral mass of the crinoid animal is encased in the aboral cup that is typically composed of 2-3 circlets of plates. The mouth and anus are on the upper or oral surface of the animal. Additional circlets of fixed arm plates and fixed interradial plates may occur above the aboral cup, making a larger calyx. Five radial plates (the uppermost circlet of aboral cup plates) are aligned with the radial water vascular canals and give rise to five arms on the oral side of the body. Each arm is an articulated series of ossicles extending outward from the body. Arms contain extensions of coelomic, nervous, water vascular, and reproductive systems and bear an ambulacral groove bordered by fingerlike tube feet, or podia (terminal extensions of the water vascular system), used in suspension feeding and respiration (see figure above). Arms may be nonbranching or branch in many different ways. All living crinoids are pinnulate, that is, they bear a small side branch (pinnule) on alternating sides of successive ossicles along the arm. In living crinoids, the pinnules bear the food-gathering tube feet. Pinnules arose in several lineages during the Paleozoic and are characteristic of all post-Palaeozoic crinoids.

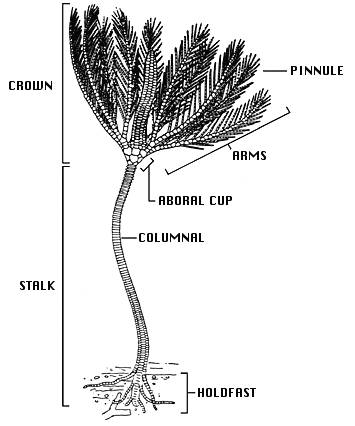
General morphology of a stalked crinoid (modified from Bather, 1900, copyright © 1998 William Ausich)
The crinoid stalk typically consists of numerous discoidal skeletal pieces called columnals, held together by ligaments and penetrated by a central canal containing coelomic and neural tissue. In most species, the stalk serves to anchor the animal permanently to the substrate via one of a variety of terminal structures, e.g., a discoidal or encrusting holdfast, rootlike radix, or grapnel. In others, such as the living isocrinids, whorls of hooklike cirri (sing., cirrus) along the stalk allow the crinoid to release its hold and crawl with its arms. Several crinoid groups, notably the comatulids, which include the only living shallow-water crinoids, have lost the stalk. Comatulids anchor via numerous cirri that arise from the retained topmost columnal (the centrodorsal).
Discussion of Phylogenetic Relationships
Traditionally, crinoids were subdivided into four subclasses: the Paleozoic Camerata, Inadunata, and Flexibilia, and the post-Paleozoic Articulata. However, a fifth subclass, the Echmatocrinea, was added in Moore & Teichert (1978). Phylogenetic relationships among these subclasses have long been poorly understood, with the exception of Flexibilia and Articulata derived from the cladid inadunates. Crinoid phylogeny and its implied classification are now the subject of intensive research and somewhat differing opinions based on differing assumptions of the crinoid outgroup and calyx plate homologies that yield different phylogenies.
Simms & Sevastopulo (1993) recognized that three major groups of crinoids were already distinct by the Lower to Middle Ordovician: the Camerata, Disparida, and Cladida. They recognized the Camerata as traditionally understood. However, they followed the suggestions of Kelly (1982, 1986) and disregarded the Inadunata as a group, because the disparids and cladids were separate, unrelated lineages. The flexibles and articulates both evolved from the cladids, so Simms and Sevastopulo (1993) placed these within the Cladida. Phylogenetic relationships in Simms and Sevastopulo (1993) were based on a limited number of characters with synapomorphies listed on a cladogram. No quantitative analyses were performed, and an emphasis was placed on Articulata characters.
Simms (1994) presented an alternative phylogeny based on a greatly revised scheme of calyx plate homologies. Rather than the radial plates (the upper plates of an aboral cup, sensu Moore & Teichert, 1978) being homologous among all crinoids and the landmark for determining all calyx plate homologies, Simms (1994) proposed that the lowest plates in the aboral cup (the infrabasal plates of three-circlet forms but the basal plates of two-circlet forms) were homologous and the homology landmarks. This substantially altered more traditional views on crinoid phylogeny, as Simms (1994) illustrated using a cladogram with inferred synapomorphies. Few workers have followed Simms (1994).
Ausich (1996a, 1997, 1998a,b) presented a phylogeny based on another alternative calyx plate homology scheme (Ausich 1996b), a differing outgroup, and parsimony-based character analyses. In this scheme, the most primitive crinoid aboral cup construction had four circlets of plates, inherited directly from rhombiferan echinoderm ancestors (Ausich 1999). An early rhombiferan echinoderm is suggested as the putative ancestor to crinoids because of shared characters among some early rhombiferans and some early crinoids. Also, Conway Morris (1993) and Ausich & Babcock (1996, 1998) do not regard Echmatocrinus from the Middle Cambrian as a crinoid. Thus, the oldest known crinoids are Early Ordovician. However, note that Sprinkle & Collins (1995), Sprinkle & Guensburg (1997), and Guensburg & Sprinkle (1997) do not follow this revision. The fourth, lowermost circlet of plates was previously unrecognized. Homologies of Ausich (1996b) only require revisions for four-circlet crinoids and disparids. The phylogeny of crinoids presented above is based on the homology scheme and PAUP parsimony analyses of Ausich (1996a, 1998a). Primitive crinoids have four circlets. Ausich followed Simms & Sevastopulo (1993) by eliminating the Inadunata and recognizing the Disparida and Cladida as separate, early crinoid lineages . The camerates are recognized by Ausich (1998a) as are the Flexibilia and Articulata. Furthermore, the camerates, flexibles, and articulates all evolved from different cladid lineages.
Evolution of Crinoidea
The four-circlet crinoids, disparids, cladids, and camerates all arose during the Early Ordovician; the flexibles arose during the Middle Ordovician, and the articulates evolved during the earliest Mesozoic. Camerates, disparids, flexibles, and cladids were extinct by the end of the Paleozoic Era, about 225 million years ago. The Articulata are the only post-Paleozoic crinoids. Either they evolved during the Permo-Triassic mass extinction or immediately thereafter.
Major evolutionary steps in the crinoid phylogeny discussed above include the following (see phylogenetic tree below): Crinoids diverged from rhombiferan echinoderms through (1) loss of pore rhombs, gonopore, and biserial brachioles; development of true arms with extension of the ambulacra; movement of the anus to the tegmen; addition of anal plates; and better pentameral symmetry. The resulting primitive crinoids were four-circlet forms constituting a low diversity, basal group (Ausich, 1998a). From this four-circlet construction, disparids arose through loss of the basal circlet (2). The cladid lineage, arose by loss of the lintel circlet (3) and gave rise to three additional very successful lineages: camerates (4) with fixed brachials and fixed interradials incorporated into the calyx, symmetrical posterior plating, and rigid plate sutures; flexibles (5) with the mouth exposed on the tegmen and loose plate sutures; and the articulates (6) with loss of the anal plate and an entoneural system enclosed within the calyx plates. The monophyletic nature of the subclass Articulata has been argued by Simms & Sevastopulo (1993).

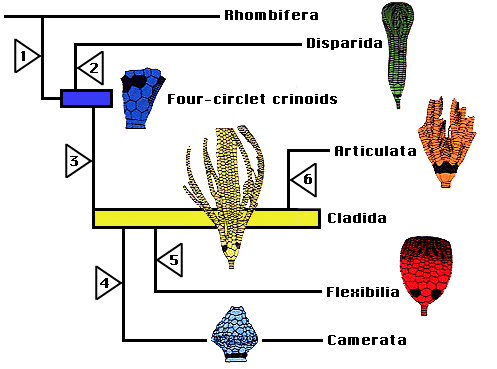
Phylogenetic hypothesis of crinoid relationships, based on Ausich (1996b, 1999).
Major Subgroups of Crinoidea
As mentioned above, Moore & Teichert (1978) recognized the four major lineages that they identified as subclasses (Camerata, Inadunata, Flexibilia, and Articulata), and they placed the single species of Echmatocrinus into its own subclass, as the primitive, basal crinoid. Revisions since 1978 include elimination of the subclass Inadunata because the disparids and cladids are quite separate lineages, elimination of Echmatocrinus as a crinoid (not universally accepted), and recognition of the oldest Early Ordovician crinoids as the initial constructional style of crinoids.
In current classification hypotheses, the Cladida, Disparida, and Camerata are regarded as subclasses (Simms and Sevastopulo 1993; Ausich 1998a). Simms and Sevastopulo (1993) group the Flexibilia and Articulata within the Cladida as a single monophyletic lineage. These relationships are not disputed by Ausich (1998a), but he proposes a partially paraphyletic classification to more accurately describe the evolutionary history of the Crinoidea. Thus, the Flexibilia and Articulata are designated as monophyletic subclasses leaving the Cladida as a paraphyletic subclass. Furthermore, the Camerata are also derived from cladids in the phylogeny of Ausich (1998a). The primitive, four-circlet crinoids are constructionally distinctive from all other crinoids and cannot be placed within any existing subclass. A new subclass is erected for these forms, however it also is paraphyletic because it gave rise to both disparids and cladids (Ausich 1998b).
Five distinct groups of articulates survive in modern seas. Their interrelationships will be treated on a separate page. The bathyal and abyssal hyocrinids have long stalks, thin discoidal columnals, and a terminal attachment disk. The bathyal and abyssal bourgueticrinids have columnals articulated by synarthries (two ligament bundles flanking a fulcral ridge) and attach via a terminal disk or rootlike radix. The bathyal isocrinids have whorls of hooklike cirri along the stalk. In the bathyal cyrtocrinids, a short stalk consists of up to two columnals, or an expanded, thickened calyx cements directly to the substrate. The comatulids, which occur from intertidal to abyssal depths, retain a stalk as postlarvae, but shed all but the topmost segment and take up a free existence as juveniles and adults.
References
Ameziane-Cominardi, N., Bourseau, J.P., Avocat, R. & Roux, M. 1990. Les crinoïdes pédonculés de Nouvelle-Caledonie: inventaire et rèflexions sur les taxons archaïques. pp. 117-124 IN: De Ridder, C. Dubois, J., Lahaye M. & Jangoux, M. (eds.) Echinoderm Research. Balkema, Rotterdam.
Ameziane-Cominardi, N. 1991. Distribution bathymetrique des pentacrines du Pacifique occidental. Essai de modelisation et d'application aux faunes du Lias. Docum. Lab. Geol. Lyon. 116. 253 p + 5 pl.
Ausich, W.I. 1980. A model for niche differentiation in lower Mississippian crinoid communities. J. Paleontol. 54:273-288.
Ausich, W.I. 1988. Evolutionary convergence and parallelism in crinoid calyx design. J. Paleontol. 62:906-916.
Ausich, W.I. 1996a. Phylogeny and classification of Ordovician Crinoidea (Echinodermata). Geol. Soc. Amer. Abstr. with Pap. 28:292.
Ausich, W.I. 1996b. Crinoid plate circlet homologies. J. Paleontol. 70:955-964.
Ausich, W.I. 1997. Calyx plate homologies and early evolutionary history of the Crinoidea. Paleont. Soc. Papers 3:289-304.
Ausich, W.I. 1998a. Early phylogeny and subclass division of the Crinoidea. J. Paleontol. 72:499-510.
Ausich, W.I. 1998b. Origin of the class Crinoidea. in: Mooi, R.; Telford, M. (Ed.) (1998). Echinoderms: San Francisco: Proceedings of the Ninth International Echinoderm Conference, San Francisco, California, USA, 5-9 August, 1996.
Ausich, W.I. 1999. Origin of crinoids, p. 237-242. In M. D. Candia Carnevali and F. Bonasara (eds.), Echinoderm Research 1998. A. A. Balkema, Rotterdam.
Ausich, W.I. & L.E. Babcock. 1996. Phylogenetic affinities of Echmatocrinus brachiatus Middle Cambrian, Canada. Paleont. Soc. Spec. Papers 8:16.
Ausich, W.I. & L.E. Babcock. 1998. The phylogenetic position of Echmatocrinus brachiatus, a probable octocoral from the Burgess Shale. Palaeontology 41:269-279.
Bather, F.A. 1900. The Echinodermata. pp. 344p. IN: Lankester, E.R. (eds.) A Treatise on Zoology, Part III. Adam and Charles Black, London.
Baumiller, T., LaBarbera, M. Woodley J.D. 1991. Ecology and functional morphology of the isocrinid Cenocrinus asterius (Linnaeus) (Echinodermata: Crinoidea): in situ and laboratory experiments and observations. Bull. Mar. Sci. 48:731-748.
Blyth Cain, J.D. 1968. Aspects of the depositional environment and paleoecology of crinoidal limestones. Scot. J. Geol. 4:191-208.
Brower, J.C. 1987. The relation between allometry, phylogeny and functional morphology in some calceocrinid crinoids. J. Paleontol. 61:999-1032.
Clark, A. H. 1915-1950. A monograph on the existing crinoids, vol. 1(parts 1, 2, 3, 4a, 4b, 4c). Bull. U. S. Natl. Mus. (82).
Clark, A. H., Clark, A. M. 1967. A monograph on the existing crinoids, 1(5). Bull. U. S. Natl. Mus. (82).
Clark, A.M. 1977. Notes on deep-water Atlantic Crinoidea. Bull. Brit. Mus. Nat. Hist., Zool. 31(4):159-186.
Conway, Morris, S. 1993. The fossil record and the early evolution of the Metazoa. Nature 361:219-225.
Donovan, S.K. 1992. Scanning EM study of the living cyrtocrinid Holopus rangii (Echinodermata, Crinoidea) and implications for its functional morphology. J. Paleontol. 66(4):665-675.
Guensburg, T.E. & J. Sprinkle. 1997. Rhombiferans are not the ancestors of crinoids. Geol. Soc. Amer. Abstr. with Prog. 29:A-341.
Heinzeller, T. & Welsch, U. 1994. Crinoidea. pp. 9-148 IN: Microscopic Anatomy of Invertebrates vol. 14. Echinodermata. Wiley-Liss, NY.
Holland, N.D., Grimmer, J.C. & Wiegmann, K. 1991. The structure of the sea lily Calamocrinus diomedeae, with special reference to the articulations, skeletal microstructure, symbiotic bacteria, axial organs, and stalk tissues (Crinoidea, Millericrinida). Zoomorphology 110:115-132.
Hyman, L. H. 1955. The Invertebrates, v. 4: Echinodermata. McGraw-Hill, New York.
Kammer, T.W. & Ausich, W.I. 1987. Aerosol suspension feeding and current velocities: distributional controls for late Osagean crinoids. Paleobiology 13:379-395.
Kelly, S.M. 1982. Origin of the crinoid orders Disparida and Cladida: Possible inadunate cup plate homologies. Third North American Paleontological Convention Proceedings, Vol. I:285-290.
Kelly, S.M. 1986. Classification and evolution of class Crinoidea. Abstracts of the Fourth North American Paleontological Converence, A23.
Lane, N.G. 1963. The Berkeley crinoid collection from Crawfordsville, Indiana. J. Paleontol. 37:1001-1008.
Macurda, D.B. & Meyer, D.L. 1974. Feeding posture of modern stalked crinoids. Nature 247:394-396.
Messing, C.G. 1987. To the Deep Reef and Beyond. Deep Ocean Society, Miami, Florida, 30p.
Messing, C.G. 1994. Comatulid crinoids (Echinodermata) of Madang, Papua New Guinea, and environs: Diversity and ecology. pp. 237-243 IN: David, B., Guille, A., Feral, J.-P. & Roux, M. (eds.) Echinoderms through Time. Balkema, Rotterdam.
Messing, C.G., Rosesmyth, M.C., Mailer, S.R. Miller, J.E. 1988. Relocation movement in a stalked crinoid (Echinodermata: Crinoidea). Bull. Mar. Sci. 42:480-487.
Meyer, D.L. 1979. Length and spacing of the tube feet in crinoids (Echinodermata) and their role in suspension feeding. Mar. Biol. 51:361-369.
Meyer, D.L. & Ausich, W.I. 1983. Biotic interactions among recent and fossil crinoids. pp. 377-425 IN: Tevesz, M.J.S. & McCall, P.L. (eds.) Biotic interactions in recent and fossil benthic communities. Plenum, NY.
Meyer, D.L. & Lane, N.G. 1976. Feeding biology of modern basketstars and some Paleozoic crinoids. J. Paleontol. 50:473-480.
Meyer, D.L. & Macurda, Jr., D.B. 1977. Adaptive radiation of the comatulid crinoids. Paleobiology 3:74-82.
Moore, R.C. & Teichert, C. (eds.) 1978. Crinoidea. Treatise on Invertebrate Paleontology, Pt. T. Echinodermata 2(1-3). Geol. Soc. Amer. & Univ. Kansas, Lawrence.
Oji, T. 1989. Growth rate of stalk of Metacrinus rotundus (Echinodermata: Crinoidea) and its functional significance. J. Fac. Sci. Univ. Tokyo (II)22:39-51.
Roux, M. 1985. Les crinoïdes pédonculés (Echinodermes) de l'Atlantique N.E.: inventaire, Écologie et biogéographie. pp. 479-489 In: L. Laubier & C. Monniot (eds.), Peuplements profonds du golfe de Gascogne. IFREMER.
Rutman, J. & Fishelson, L. 1969. Food composition and feeding behavior of shallow-water crinoids at Eilat (Red Sea). Mar. Biol. 3:46-57.
Simms, M.J. 1988. The phylogeny of post-Paleozoic crinoids. pp. 269-284 In: Paul, C.R.C. & Smith, A.B. (eds.), Echinoderm Phylogeny and Evolutionary Biology. Clarendon Press, Oxford.
Simms, M.J. 1994. Reinterpretation of thecal plate homlogy and phylogeny in the class Crinoidea. Lethaia 26:303-312.
Simms, M.J. & Sevastopulo, G.D. 1993. The origin of Articulate crinoids. Palaeontology 36(1):91-109.
Sprinkle, J. & D. Collins. 1995. Echmatocrinus revisited: still an echinoderm and probably the oldest crinoid. Geol. Soc. Amer. Abstr. with Prog. 27:A113-A114.
Sprinkle, J. & T.E. Guensburg. 1997. How the crinoid got its cup plating: Arm brachials down plus stem (penta)meres up. Geol. Soc. Amer. Abstr. with Prog. 29:A-341.
Ubaghs, G. 1953. Classe des Crinoides. pp. 658-773 In: J. Piveteau (ed.), Traité de Paléontologie 3. Masson et Cie, Paris.
Willkie, I.C., Emson, R.H., Young, C.M. 1993. Smart collagen in sea lilies. Nature 366:519-520.
Title Illustrations

Scientific Name | Onychocrinus exsculptus |
---|---|
Location | Indiana |
Comments | Cladida |
Specimen Condition | fossil |
Image Use |
![]() |
Copyright |
© 1997 William I. Ausich
![]() |
Scientific Name | Oxycomantus bennetti |
---|---|
Location | "Vanessa's Reef," Kimbe Bay, New Britain, Papua New Guinea |
Specimen Condition | Live Specimen |
Source | Oxycomantus bennetti |
Source Collection | Flickr |
Image Use |
![]() |
Copyright | © 2008 erikschlogl |
Scientific Name | Neocrinus decorus |
---|---|
Location | Grand Bahama Island, 420m |
Comments | Isocrinidae |
Image Use |
![]() |
Copyright |
© 1997 Charles G. Messing
![]() |
About This Page
William I. Ausich
Ohio State University, Columbus, Ohio, USA
Charles G. Messing
Nova Oceanographic Center, Dania, Florida, USA
Correspondence regarding this page should be directed to William I. Ausich at
Page copyright © 1998 William I. Ausich and Charles G. Messing
Page: Tree of Life
Crinoidea. Sea lilies and feather stars.
Authored by
William I. Ausich and Charles G. Messing.
The TEXT of this page is licensed under the
Creative Commons Attribution License - Version 3.0. Note that images and other media
featured on this page are each governed by their own license, and they may or may not be available
for reuse. Click on an image or a media link to access the media data window, which provides the
relevant licensing information. For the general terms and conditions of ToL material reuse and
redistribution, please see the Tree of Life Copyright
Policies.
- First online 01 April 1998
- Content changed 21 April 1998
Citing this page:
Ausich, William I. and Charles G. Messing. 1998. Crinoidea. Sea lilies and feather stars. Version 21 April 1998. http://tolweb.org/Crinoidea/19232/1998.04.21 in The Tree of Life Web Project, http://tolweb.org/